
R&B Advanced Cardiovascular Research

Introduction
Cardiovascular diseases are one of the main reasons behind deaths according to recent reports. Approximately, 92.1 million American adults have at least one type of cardiovascular disease [1]. 30% of adults with an existing cardiovascular condition suffer from heart disease. For the last century, cardiovascular diseases have been considered the main cause of death in the United States. Depending on the severity and type of the condition, treatment or countermeasure options may vary. Altering lifestyle habits, such as weight control, increasing physical activity, quitting the consumption of tobacco products, moderating alcohol intake, and decreasing saturated fat and sodium in the diet, are shown to be beneficial to improving cardiovascular health. Moreover, assistive medication for high blood pressure or high cholesterol is also beneficial. For people who have been diagnosed with severe conditions, lifestyle changes, and assistive medication may not be enough. Heart transplantation could be considered the best possible treatment. Yet, the ratio of available heart donors to people on the transplantation waitlist is not promising [2]. The World Health Organization’s “Atlas of Heart Disease and Stroke [3]” foresees a grim future for the World’s population. One of the predictions of the report is that by 2030 globally 32.5 % of all deaths would be caused by cardiovascular diseases. The report also plants hope by stating that cardiovascular diseases could be prevented considering research conducted for the last 50 years. Therefore, there is an imminent need for the development of new treatments for cardiovascular diseases and the development and testing of proposed future treatments. The development of a novel Cardiovascular Performance Benchmark platform (CPSP) would be a perfect candidate to meet the requirements of such a need.
Goal
Equipping the Healthcare Industry to Achieve Innovative Design Breakthrough
01. Design and Development of a 3D Printing Machine to Manufacture Customized Silicone Heart Valve
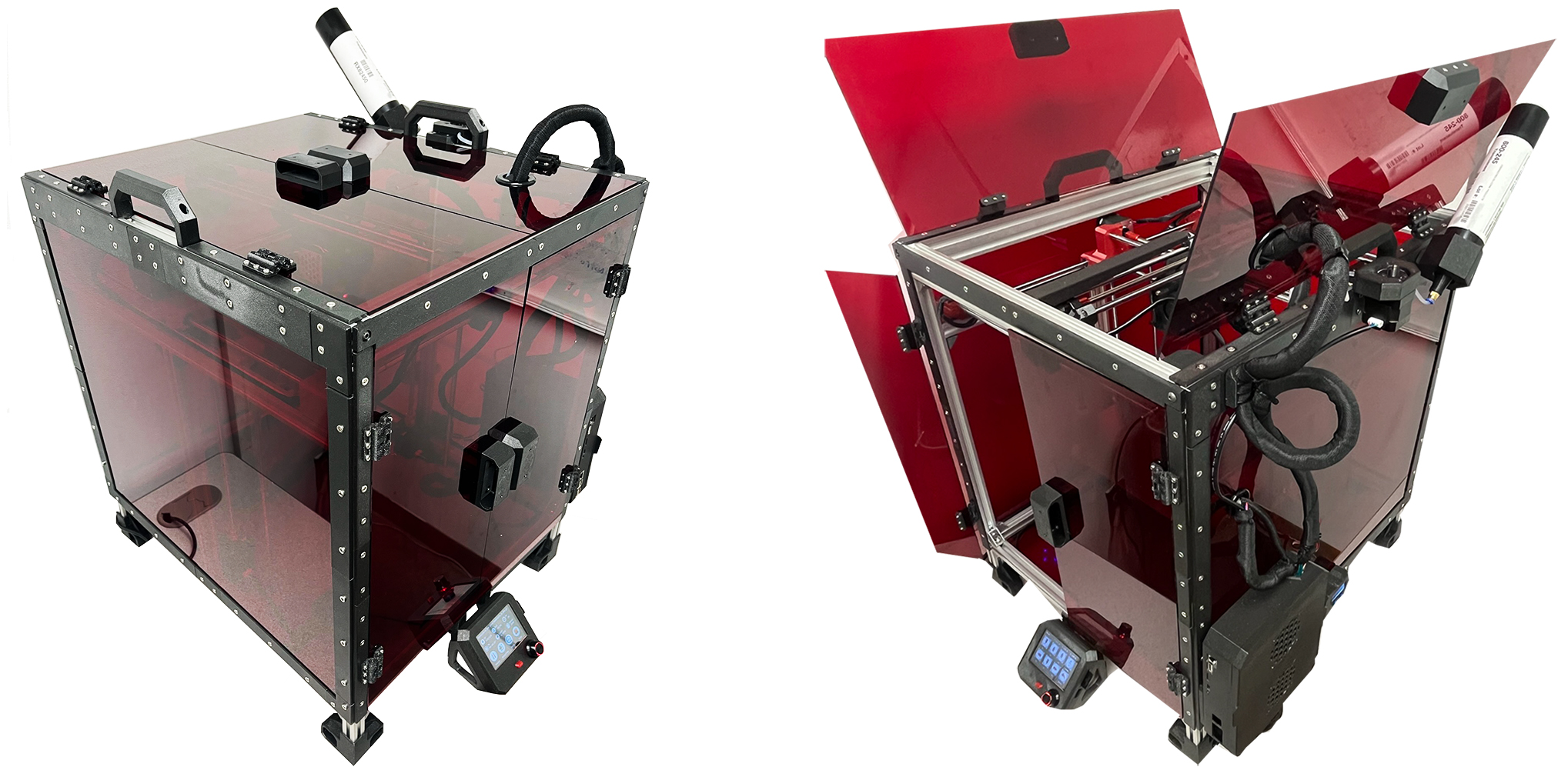
Fig.2 3D silicone printing machine final product (Ertas et al., 2023).
3D Printing of Silicone Heart Valve: A Major Breakthrough
Researchers studying additive manufacturing are very interested in new materials and procedures suitable for 3D printing applications [4, 5]. Custom-made heart valves can be created as functional implants for patients by precisely recreating the geometry of the aortic root region using 3D scanning technology, computer-aided design, and 3D printing.
Since silicone is known to be biocompatible, it is a good contender to be utilized as the material for heart valve fabrication. Despite being biocompatible, silicone heart valve components are not currently intended for use in human tissue. They will initially function as a model to replicate the actions of bioprosthetic valves. 3D-printed silicone heart valves may eventually be modified for use as implantable artificial heart valves [6].
However, there are difficulties with 3D printing silicone. Silicone is an elastomer, and unlike thermoplastics, which can melt and then solidify again, silicone cannot be melted once it has solidified. Furthermore, silicone is difficult to cure in its pure state.
Despite numerous difficult challenges with silicone printing, our research team has successfully manufactured a 3D-printed silicon heart valve, which is a breakthrough in regenerative medicine and gives hope to those who suffer from heart problems (please watch the above video).
02. Mock Circulation Loops (MCL) to Test Heart Valve Functionality
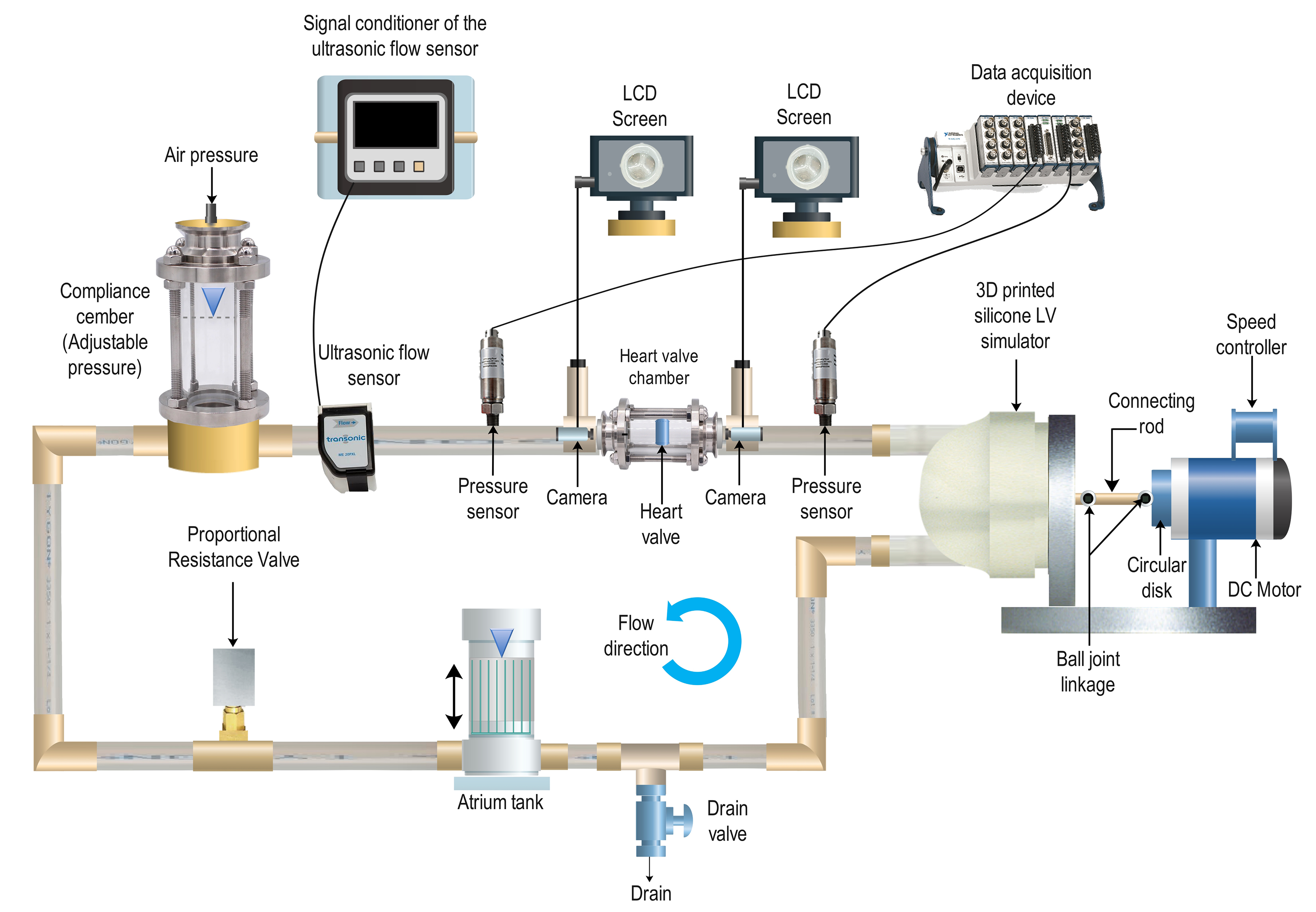
Fig. 3 Prototyped MCL system (adapted from Baturalp and Ertas, 2015).
03 Cardiovascular Performance Simulation Platform (CPSP)
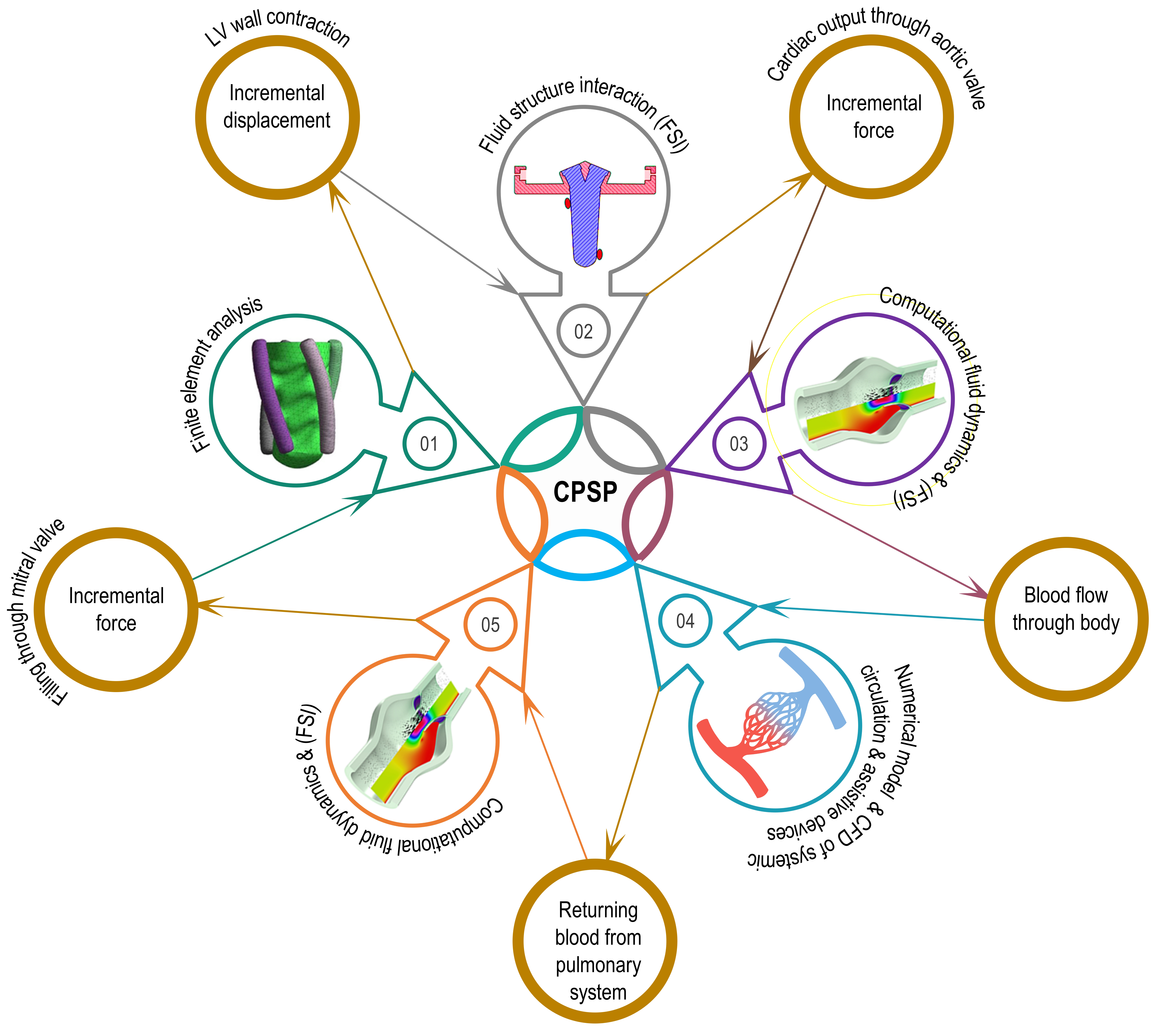
Fig. 4 Proposed Cardiovascular Performance Simulation Platform. (adapted from Gulbulak and Ertas, 2019).
04. Development of Left Ventricular Assistance Devices (LVAD)
The results of this study will be a stepping stone for researchers all around the globe who have been working on a better understanding of cardiovascular diseases and effective long-term cardiovascular treatments by means of either clinical, computational, or experimental methods. CPSP will accelerate the mentioned research efforts by offering a benchmark strategy that will be as physiologically accurate as possible.
References
-
Benjamin, E. J., et al., “Heart Disease and Stroke Statistics—2017 Update: A Report From the American Heart Association.” Circulation 135, no. 10 (2017): e146–e603. doi:10.1161/CIR.0000000000000485, Available at http://circ.ahajournals.org/lookup/doi/10.1161/CIR.0000000000000485
-
Califano, S., Pagani, F. D., and Malani, P. N. “Left Ventricular Assist Device-Associated Infections.” Infectious Disease Clinics of North America 26, no. 1 (2012): 77–87. doi:10.1016/j.idc.2011.09.008, Available at http://www.ncbi.nlm.nih.gov/pubmed/22284377
-
“WHO | The Atlas of Heart Disease and Stroke.” WHO (2010): Available at http://www.who.int/cardiovascular_diseases/resources/atlas/en/
-
Radu, M.; Iuliana, M.B., Laurentiu, C.C.; Razvan, E., Alexandru, P., Andrei, T.C., Gabriela, S., Elisa, P., and Teodor, B. (2021). In Vitro Physical-Chemical Behaviour Assessment of 3D-Printed CoCrMo Alloy for Orthopaedic Implants. Metals, 11(6), 857; https://doi.org/10.3390/met11060857
-
Khaja M.; Syed, H.M., Wadea, A., Hisham A., and Abdul S. (2021). Feasibility Study of the Cranial Implant Fabricated without Supports in Electron Beam Melting. Metals, 2021, 11(3), 496; https://doi.org/10.3390/met11030496
-
Coulter, F. B., Schaffner, M., Faber, J. A., Rafsanjani, A., Smith, R., Appa, H., Zilla, P., Bezuidenhout, D., Studart, A. R. (2019). Bioinspired Heart Valve Prosthesis Made by Silicone Additive Manufacturing. Matter, Vol.1, Issue 1, 2019, pp. 266-279.
Related Published Articles
Ertas, A., Erik Farley-Talamantes, H. Saker, A. Rajan, C. Lamb, D. Flores, B. Norton. Innovative Approach to Design and Development of a 3D Silicone Printing Machine Using Transdisciplinary Integrated Design Tools. Transdisciplinary Journal of Engineering & Science, Vol. 14, pp. 19-63, 2023.
Gulbulak, U., Gecgel, O., and Ertas, A. A Deep Learning Framework to Approximate the Geometric Orifice and Coaptation Area of Polymeric Heart Valves Under Time-Varying Transvalvular Pressure. Journal of the Mechanical Behavior of Biomedical Materials, 2021, 117:104371. doi: 10.1016/j.jmbbm.2021.104371.
Gulbulak, U., Ertas, A., Pavelka, T., Baturalp, T. The Effect of Fundamental Curves on Geometric Orifice Area of Polymeric Bioprosthetic Heart Valves. Journal of the Mechanical Behavior of Biomedical Materials, 112, 104039. https://doi.org/10.1016/j.jmbbm.2020.104039.
Gulbulak, U., Ertas, A. Finite Element Driven Design Domain Identification of a Beating Left Ventricular Simulator. Bioengineering, 2019, 6, 83; doi:10.3390/bioengineering6030083
Gulbulak U., and Ertas, A., and Students from Additive Manufacturing class. Boosting Just-in-Time Supply Chain Innovation through Additive Manufacturing: A Transdisciplinary Educational Experience. Transdisciplinary Journal of Engineering & Science, Vol. 10, pp. 199-223, 2019. doi: 10.22545/2020/00137
Pavelka, T., Gulbulak, U., Baturalp, T., Ertas, A. Performance Evaluation of a Mock Circulation Loop with Bioprosthetic Heart Valves and a Beating Left Ventricle Simulator. Rice University, Houston, Texas, "Gold Coast Undergraduate Research Symposium," National, peer-reviewed/refereed. (2019).
Baturalp, T. B., Ertas, A. State of the Art Mock Circulation Loop and a Proposed Novel Design (pp. 23-29, 2015). Proceedings of the International Conference on Biomedical Engineering and Science (BIOENG'15).